Columbia University
Irving Medical Center
Neurological Institute
710 West 168th Street, 3rd floor
(212) 305-1818


TaubCONNECT Research Perspective:
June 2025
APOE and Alzheimer's Disease and Related Dementias Risk Among 12,221 Hispanics/Latinos
A Human Brain Map of Mitochondrial Respiratory Capacity and Diversity
ANXA11 Biomolecular Condensates Facilitate Protein-Lipid Phase Coupling on Lysosomal Membranes
Axonal Transport of CHMP2b Is Regulated by Kinesin-Binding Protein and Disrupted by CHMP2bintron5
Sleep Genetics and Cognitive Changes over Time: The Moderating Effect of Age and the Role of Brain
Emerging Roles for Tubulin PTMs in Neuronal Function and Neurodegenerative Disease
Inflammatory Biomarkers Profiles and Cognition Among Older Adults
Synaptic and Cognitive Impairment Associated with L444P Heterozygous Glucocerebrosidase Mutation
Regulation of Synapse Density by Pumilio RNA-Binding Proteins
CD33 and SHP-1/PTPN6 Interaction in Alzheimer's Disease
Cellular Communities Reveal Trajectories of Brain Ageing and Alzheimer's Disease
Epigenetic and Genetic Risk of Alzheimer Disease from Autopsied Brains in two Ethnic Groups
Multi-Omic Analysis of Huntington's Disease Reveals a Compensatory Astrocyte State
Design and Methods of the Early Age-Related Hearing Loss Investigation Randomized Controlled Trial
Updated Safety Results From Phase 3 Lecanemab Study in Early Alzheimer's Disease
The Broken Alzheimer's Disease Genome
Rare Genetic Variation in Fibronectin 1 (FN1) Protects Against APOEε4 in Alzheimer's Disease
Cell Subtype-Specific Effects of Genetic Variation in the Alzheimer's Disease Brain
Diet, Pace of Biological Aging, and Risk of Dementia in the Framingham Heart Study
A Comparative Study of Structural Variant Calling in WGS from Alzheimer's Disease Families
Glucocorticoid Stress Hormones Stimulate Vesicle-Free Tau Secretion and Spreading in the Braint
The Effects of Insufficient Sleep and Adequate Sleep on Cognitive Function in Healthy Adults
ZCCHC17 Modulates Neuronal RNA Splicing and Supports Cognitive Resilience in Alzheimer's Disease
Effects of Lithium on Serum Brain-Derived Neurotrophic Factor in Alzheimer's Patients with Agitation
2023 Taub Institute Grants for Emerging Research (TIGER) Awardees!
Rie1 and Sgn1 Form an RNA-Binding Complex that Enforces the Meiotic Entry Cell Fate Decision
Memory and Language Cognitive Data Harmonization Across the United States and Mexico
Education as a Moderator of Help Seeking Behavior in Subjective Cognitive Decline
Multicellular Communities are Perturbed in the Aging Human Brain and Alzheimer's Disease
The Neuropathological Landscape of Hispanic and non-Hispanic White Decedents with Alzheimer Disease
The Early-Onset Alzheimer's Disease Whole-Genome Sequencing Project: Study Design and Methodology
Polygenic Risk Score Penetrance & Recurrence Risk in Familial Alzheimer Disease
High School Quality is Associated with Cognition 58 Years Later
Glucocorticoid-Driven Mitochondrial Damage Stimulates Tau Pathology
A Global View of the Genetic Basis of Alzheimer Disease
ARIA in Patients Treated with Lecanemab (BAN2401) in a Phase 2 Study in Early Alzheimer's Disease
Microglia Reactivity Entails Microtubule Remodeling from Acentrosomal to Centrosomal Arrays
Genuine Selective Caspase-2 Inhibition with new Irreversible Small Peptidomimetics
Cell Type-Specific Changes Identified by Single-Cell Transcriptomics in Alzheimer's Disease
Brain Aging Among Racially and Ethnically Diverse Middle-Aged and Older Adults
First Place: Neuroproteasome Localization and Dysfunction Modulate Pathology in Alzheimer's Disease
Clearance of an Amyloid-Like Translational Repressor is Governed by 14-3-3 Proteins
Diet Moderates the Effect of Resting State Functional Connectivity on Cognitive Function
Retromer Deficiency in Tauopathy Models Enhances the Truncation and Toxicity of Tau
Progranulin Mutations in Clinical and Neuropathological Alzheimer's Disease
Wolframin is a Novel Regulator of Tau Pathology and Neurodegeneration
Homotypic Fibrillization of TMEM106B Across Diverse Neurodegenerative Diseases
Correlation of Plasma and Neuroimaging Biomarkers in Alzheimer's Disease
Tubulin Tyrosination Regulates Synaptic Function and is Disrupted in Alzheimer's Disease
The Penalty of Stress - Epichaperomes Negatively Reshaping the Brain in Neurodegenerative Disorders
The Neuronal Retromer can Regulate Both Neuronal and Microglial Phenotypes of Alzheimer's Disease
Deep Learning Improves Utility of Tau PET in the Study of Alzheimer's Disease
Age of Onset of Huntington's Disease in Carriers of Reduced Penetrance Alleles
Caspase-9: A Multimodal Therapeutic Target With Diverse Cellular Expression in Human Disease
Midlife Vascular Factors and Prevalence of Mild Cognitive Impairment in Late-Life in Mexico
The Association Between Sex and Risk of Alzheimer's Disease in Adults with Down Syndrome
Marked Mild Cognitive Deficits in Humanized Mouse Model of Alzheimer's-Type Tau Pathology
Rapid ATF4 Depletion Resets Synaptic Responsiveness after cLTP
Polygenic Risk Score for Alzheimer's Disease in Caribbean Hispanics
Recognition Memory and Divergent Cognitive Profiles in Prodromal Genetic Frontotemporal Dementia
The Microtubule Cytoskeleton at the Synapse & The Synaptic Life of Microtubules
Optimizing Subjective Cognitive Decline to Detect Early Cognitive Dysfunction
The AD Tau Core Spontaneously Self-Assembles and Recruits Full-Length Tau to Filaments
Olfactory Impairment is Related to Tau Pathology and Neuroinflammation in Alzheimer's Disease
Pathogenic Role of Delta 2 Tubulin in Bortezomib-Induced Peripheral Neuropathy
2: HDAC Inhibitors Engage MITF and the Disease-Associated Microglia Signature to Enhance Amyloid β Uptake
3: The Role of Alpha-Synuclein in Synucleinopathy: Impact on Lipid Regulation at Mitochondria-ER Membranes
4: Associations Between Hearing Loss and Dementia in a Large Electronic Health Record System
Early Proteasome Downregulation and Dysfunction Drive Proteostasis Failure in Alzheimer's Disease
![]() | ![]() | ![]() | ||
Shan Jiang, PhD | Malavika Srikanth, PhD | Natura Myeku, PhD |
In this study, recently published in BRAIN, we investigated a fundamental yet underexplored state of the ubiquitin–proteasome system (UPS) in Alzheimer’s disease (AD). We uncovered an early, intrinsic collapse in proteasome function that precedes overt protein tau aggregation, positioning the UPS as an early casualty and active contributor to proteostasis collapse in AD. Using human postmortem brain tissue, we combined proteasome activity assays and proteomics analyses to reveal that both 26S and 20S proteasomes are catalytically impaired in AD, even after purification, indicating structural deficits within the complexes themselves. Proteomic profiling revealed a marked depletion of core 19S and 20S subunits, as well as essential assembly chaperones, particularly in neuron-rich grey matter. Notably, 26S proteasomes from AD brains co-purified with aggregation-prone proteins like tau, α-synuclein, and p62/SQSTM1, suggesting that they are not only dysfunctional but also physically trapped within insoluble aggregates.
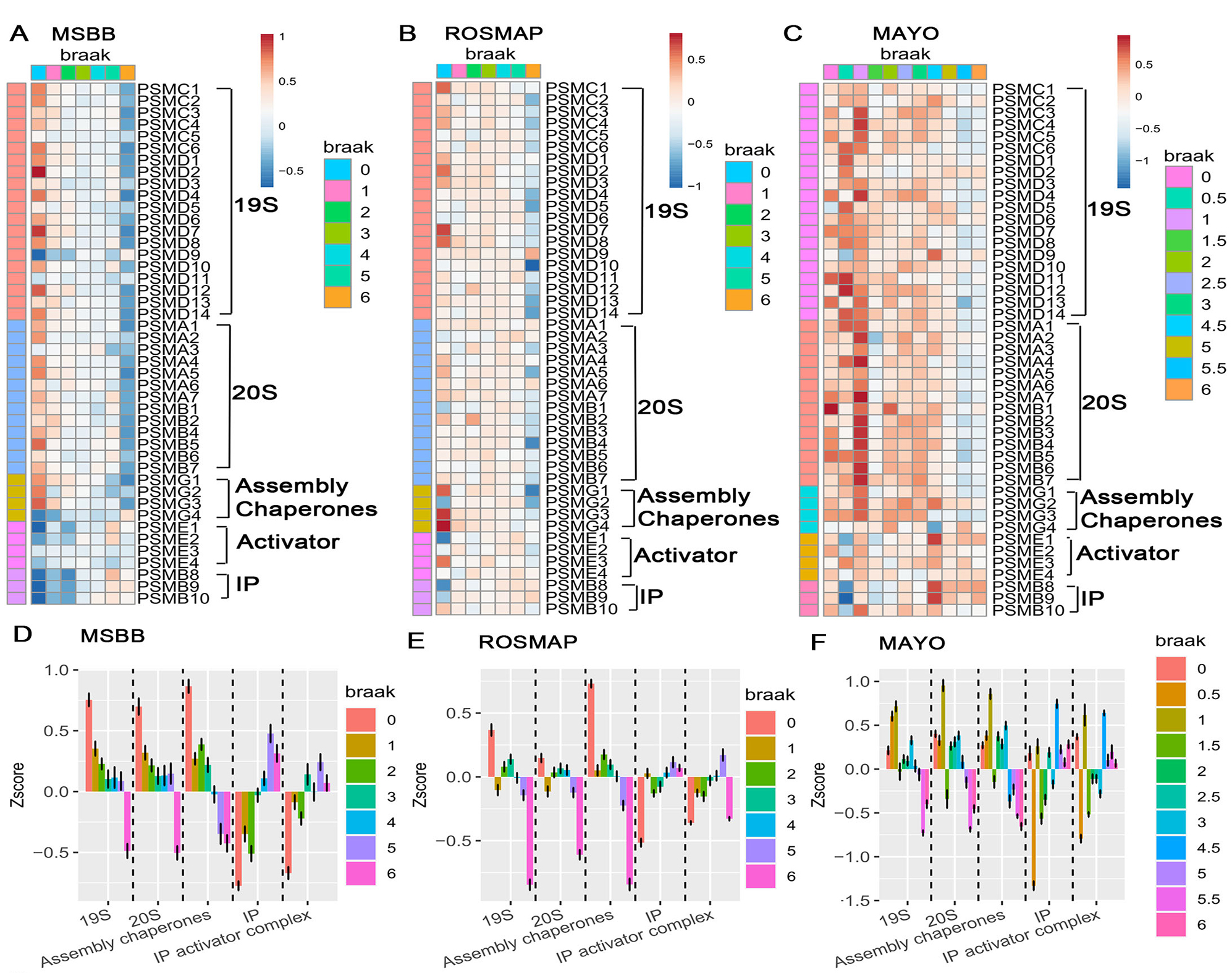
Figure 4. Progressive downregulation of constitutive proteasome subunits and differential responses of immunoproteasome across multiple AD cohorts. (A–C) Heatmaps showing the normalized expression (Z-scores) of constitutive proteasome subunits, assembly chaperones, and immunoproteasome (IP) components across increasing Braak stages for three independent cohorts of bulk-RNAseq datasets: (A) MSBB, (B) ROSMAP, and (C) Mayo Clinic Study of Aging. Each column represents a sample ordered by Braak stage (0-VI), while rows depict individual proteasome-related genes. Warmer colors (reds) indicate higher relative expression, and cooler colors (blues) indicate lower expression. A clear trend emerges where constitutive proteasome subunits (19S and 20S) and assembly chaperones decline with the advancing Braak stage, whereas immunoproteasome subunits show less consistent changes. (D–F) Boxplots of Z-scores for aggregated proteasome complexes and factors, 19S, 20S, assembly chaperones, immunoproteasome (IP), and IP activator complexes—grouped by Braak stage in (D) MSBB, (E) ROSMAP, and (F) Mayo cohorts. Each colored point or bar corresponds to a specific Braak stage. As Braak stage increases, 19S and 20S components consistently show a downward trend, while immunoproteasome and IP activator complexes remain relatively stable or exhibit compensatory changes.
The transcriptomics analyses of bulk RNA-seq datasets from three large AD cohorts (MSBB, ROSMAP, and Mayo) revealed a striking and progressive downregulation of constitutive proteasome subunit genes beginning at the earliest Braak stages, well before the appearance of tau pathology. These transcriptional deficits were further validated by snRNA-seq of two independent ROSMAP datasets, which revealed that this proteasome gene suppression is largely neuron-specific. In contrast, glial cells maintained or even upregulated proteasome-related transcripts, particularly those associated with the immunoproteasome, suggesting a cell–type–specific adaptive response to proteotoxic stress.
We then asked why neurons fail to mount a compensatory proteasome response. Under normal conditions of proteasome stress, cells activate the transcription factor NFE2L1 (Nrf1), which drives de novo synthesis of proteasome subunits through the “bounce-back” response. However, in AD brains, we observed that while Nrf1 transcript levels are elevated, its protein remains sequestered in the cytoplasm and fails to translocate to the nucleus, preventing effective activation of proteasome gene expression. We developed an “Nrf1 resist score” that quantifies this regulatory breakdown across disease stages, revealing that the disconnect between Nrf1 levels and proteasome gene expression intensifies as AD progresses. This nuclear translocation defect was further confirmed by subcellular fractionation and immunoblotting of human brain samples.
Our findings indicate that proteasome dysfunction is not merely a downstream consequence of AD pathology but may be a critical upstream event that promotes protein aggregation and neuronal vulnerability. Targeting proteasome function or restoring Nrf1 signaling represents a promising therapeutic strategy for preserving proteostasis in the aging brain.
Natura Myeku, PhD
Associate Professor of Pathology and Cell Biology
nm2631@cumc.columbia.edu

![]() | ![]() | |
Verena Haage, PhD | Philip L. De Jager, MD, PhD |
Disease-associated microglia (DAM) have been widely implicated in neurodegeneration, yet their precise role and regulatory mechanisms in the human brain remain incompletely defined. To address this, our team from the Taub Institute and the Center for Translational & Computational Neuroimmunology (CTCN), led by first author Dr. Verena Haage, in collaboration with colleagues from the Ludwig Center for Research on Neurodegeneration, developed a pharmacological approach to model human DAM in vitro—work recently published in Brain, Behavior, and Immunity.
Using an in silico screening strategy, we prioritized two HDAC inhibitors, Vorinostat and Entinostat, which reproducibly engage key components of the human DAM transcriptional signature in microglia-like cells. These compounds not only induced expression of canonical DAM markers—including CD9, LPL, and SPP1—but also significantly upregulated MITF, a transcription factor recently proposed as a regulator of DAM-associated programs. Importantly, HDAC inhibitor-treated cells exhibited selective functional changes: enhanced uptake of amyloid β and dextran, reduced phagocytosis of bacterial particles, and attenuation of MCP-1 secretion in response to inflammatory stimuli. These findings were validated across two distinct human microglial model systems: the HMC3 microglia-like cell line and iPSC-derived microglia.
By providing a scalable and reproducible method to induce DAM-like states in human cells, our work advances the experimental toolkit for dissecting DAM biology. Moreover, our results support the emerging view that DAM signatures reflect at least two distinct transcriptional programs, underscoring the complexity of microglial polarization in neurodegenerative disease contexts. This platform offers new opportunities to mechanistically interrogate DAM function and to explore the translational potential of pharmacologically modulating microglial states.
Philip L. De Jager, MD, PhD
Weil-Granat Professor of Neurology(in The Taub Institute)
pld2115@cumc.columbia.edu

![]() | ![]() | ![]() | ||
Peter Barbuti, PhD | Estela Area Gomez, PhD | Serge Przedborski, MD, PhD |
While alpha-synuclein (αSyn) dysfunction has long been recognized as central to the pathogenesis of synucleinopathies, the precise mechanisms linking αSyn to neuronal vulnerability remain incompletely understood. In our study, led by first author Dr. Peter Barbuti and conducted in collaboration with Dr. Estela Area-Gomez and colleagues from the Taub Institute and the Ludwig Center for Research on Neurodegeneration, we investigated the intersection of αSyn biology and lipid homeostasis, with a particular focus on mitochondria-associated ER membranes (MAMs), key subcellular domains regulating lipid metabolism.
As reported in npj Parkinson’s Disease, through lipidomic profiling of postmortem brain samples from individuals with Parkinson’s disease (PD), multiple system atrophy (MSA), and healthy controls, we identified region- and disease-specific disruptions in sterols, phospholipids, and sphingolipids. The substantia nigra pars compacta (SNpc) of PD patients exhibited a distinct lipid signature, including elevated cholesteryl esters, reduced ceramides, and accumulation of polyunsaturated phosphatidylcholine and phosphatidylserine species—alterations only partially mirrored in MSA striatum. These findings suggest that αSyn-driven lipid dyshomeostasis may underlie selective regional vulnerability in PD.
To probe the mechanistic basis for these observations, we utilized human iPSC-derived neurons expressing varying levels of αSyn. These experiments revealed that αSyn localizes to MAM domains and regulates phosphatidylserine metabolism in a dosage-dependent manner. Notably, increased αSyn expression, as occurs with SNCA duplication, perturbs MAM lipid composition, disrupts sphingolipid and phospholipid homeostasis, and impairs PS decarboxylation—phenotypes consistent with lipid alterations observed in PD brain tissue. Together, these data implicate αSyn dosage and MAM dysfunction as key drivers of lipid dysregulation in synucleinopathy and highlight lipid metabolism as a promising axis for biomarker discovery and therapeutic intervention.
Serge Przedborski, MD, PhD
Page and William Black Professor of Neurology (in Pathology and Cell Biology and Neuroscience)
sp30@cumc.columbia.edu

Associations Between Hearing Loss and Dementia in a Large Electronic Health Record System
![]() | ![]() | |
James Noble, MD, MS | Justin Golub, MD, MS |
In the current study, led by Dr. Justin Golub (Columbia Otolaryngology–Head and Neck Surgery), we examined the association between audiometric hearing loss and dementia using a large, multi-institutional electronic health record (EHR) dataset. Leveraging data from nearly 32,000 adult patients who underwent formal audiometric testing at NYP/Columbia and Weill Cornell medical centers, we were able to assess hearing loss comprehensively through pure tone average, word recognition score, and speech reception threshold—three objective measures not consistently captured in national epidemiologic datasets.
As recently reported in The Laryngoscope, our findings demonstrated that greater degrees of hearing loss, regardless of the metric used, were associated with significantly increased odds of dementia, even after controlling for key demographic and clinical confounders, including age, sex, cardiovascular risk, and study site. Notably, the relationship was consistent across different definitions of dementia, including diagnosis codes and medication use, underscoring the robustness of the association. The magnitude of risk observed—particularly among individuals with moderate to severe hearing loss—highlights the potential clinical significance of these findings.
While observational by design, this study reinforces prior work identifying hearing loss as a modifiable risk factor for cognitive decline and dementia. Furthermore, by demonstrating the feasibility and scientific value of leveraging large-scale EHR data, this work provides a framework for future longitudinal and interventional studies aimed at understanding the mechanistic pathways linking auditory and cognitive health, as well as evaluating the potential cognitive benefits of timely hearing loss treatment.
James Noble, MD, MS
Professor of Neurology (in the Taub Institute and the Gertrude H. Sergievsky Center)
jn2054@cumc.columbia.edu
